Architected Materials and Structures
Lattice Metamaterials
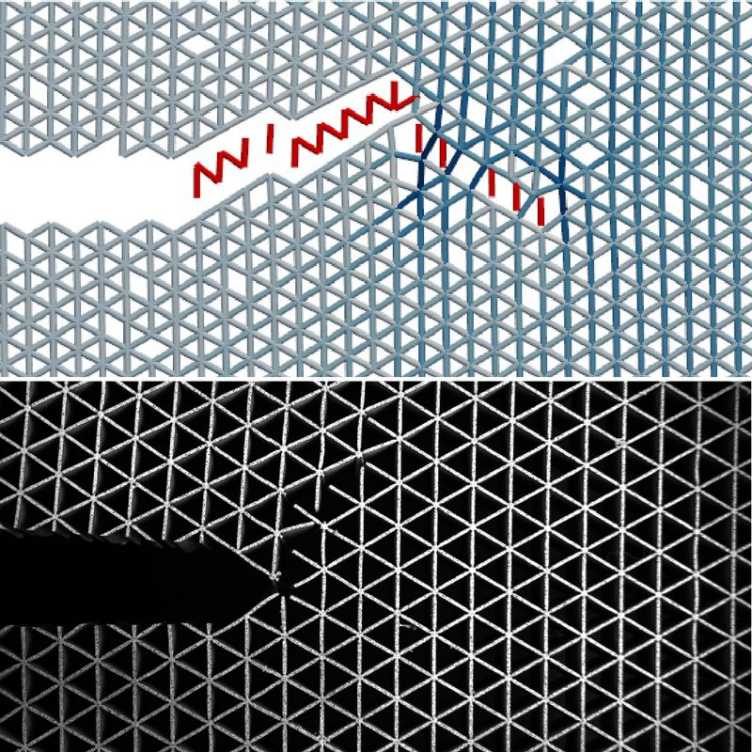
Architected materials are lattice-like structures engineered through the repetition of unit cells. Manipulating their topology grants the ability to attain tailored, specific properties dissimilar from those of the underlying material, such as negative Poisson’s ratio, energy absorption, or toughening. Despite their promising potential, one of the primary challenges of architected materials lies in the complexity of accurately predicting their mechanical response, hindering their broader application in engineering solutions.
Our work combines experimental and numerical approaches to unravel the local fracture behaviour of 2D and 3D lattices. We develop numerical models to predict damage initiation and propagation at the strut level and tune characterisation techniques to monitor the crack propagation and energy-to-failure in 3D-printed lattices. Moreover, we investigate how local perturbations in the geometrical repetition of the unit cell, whether by design or arising from the 3D printing process itself, impact the crack path and the work to destructive failure. Ultimately, our interdisciplinary approach aims to unlock new design strategies to manufacture architected materials with controlled crack propagation and delayed destructive failure.
Topologically Interlocked Structures
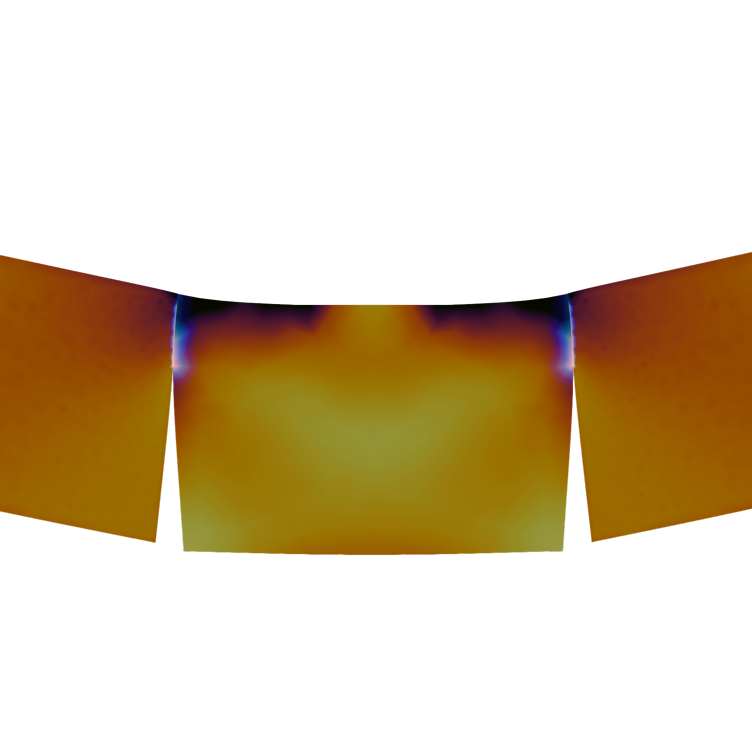
Topologically interlocked structures (TIS) are assemblies of specially shaped blocks that withstand loads by transmitting forces to neighboring blocks solely through contact and friction, without the use of adhesives. These structures exhibit unique properties, including high strength, energy dissipation, crack arrest abilities, and work-to-failure. However, the failure mechanisms of TIS are complex and extremely challenging to predict, which impedes their widespread application in engineering practice.
In our work, we aim to establish a better understanding of the failure mechanisms of TIS. To this end, we develop numerical methods to improve the modeling of these structures, including specifically tailored techniques such as the level-set discrete element method. Additionally, we research the scaling properties of TIS and determine their maximal performance limit. We also explore options for enhancing the performance of TIS, for example, by designing hierarchical surfaces. Ultimately, our research contributes to better predictability of the failure mechanics of TIS and strengthens their use in engineering applications.